Prof. Dr.-Ing. Christian Hasse,
Head of STFS
Simulation has become the third scientific pillar, alongside theory and experiment.
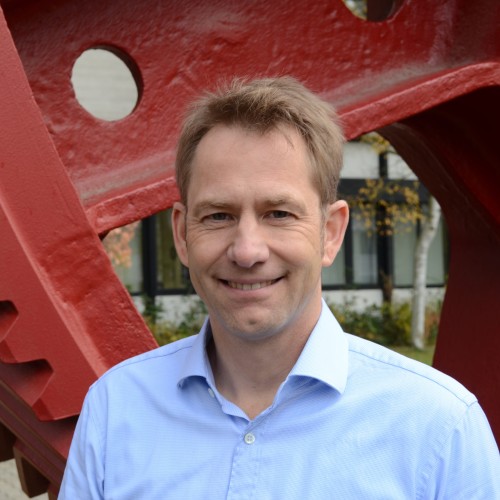
Our research at the STFS can be arranged in four dimensions:
- Configurations
- Energy Carriers
- Theory and Physical Modeling
- Computational Methods
These are presented in more detail.
We investigate configurations ranging from simple laminar, freely propagating flames to complex technical combustion chambers. As part of this, we examine laboratory flames which are designed for the targeted analysis of physical effects during combustion. Thus, we bridge the gap between basic research and application.
The energy carriers we investigate range from gaseous hydrogen to iron and aluminum powder, all of which come with their own challenges.
At the STFS we conduct research into flamelet theory, apply large-eddy simulations (LES) and develop corresponding models, for example models for artificial flame thickening for turbulence-chemistry interaction.
Computational methods are essential for our research in order to be able to examine our models in different configurations under consideration of the various fuels. The focus here is on high-performance computing on state-of-the-art supercomputers and the subsequent analysis and visualization of the simulation results.
Our research dimensions are integrated into various research areas, which also form the basis of our internal organizational structure. However, the areas do not form sharp boundaries, but overlap in many aspects and blend into one another. For example, the models developed in fundamental research on laminar and turbulent flames are also used in simulations of aircraft engines and the software we use for the simulation of multiphase and multicomponent flows is identical.
Software
At STFS, we use various software tools to develop, validate and apply models. These are primarily simulation software packages for numerical flow simulations, which offer options for simulating multiphase and multicomponent reactive flows. Our in-house developed software ULF (Universal Laminar Flame Solver) is mainly used in model development and model validation for the numerical solution of 0D, 1D and 2D problems (e.g. for the solution of flamelet equations and balance equations of generic flame configurations). We use the newly developed GPU-accelerated spectral element Navier-Stokes solver nekRS as the basis for model development by means of direct numerical simulations (DNS) of turbulent reactive flows.
The most widely used software at STFS is the open source program OpenFOAM®. It is used for laminar direct numerical simulations in model development and model validation, as well as for coupling our models with scale-resolving coarse-structure simulations.
We also use commercial CFD software in cooperation with industrial partners and for certain technical applications.
Extensive computing capacities are available with the infrastructure of , which includes the Lichtenberg high-performance computer of TU Darmstadt and the CLAIX high-performance computer of RWTH Aachen University. NHR4CES
We also operate our own computing cluster for smaller-scale simulations.